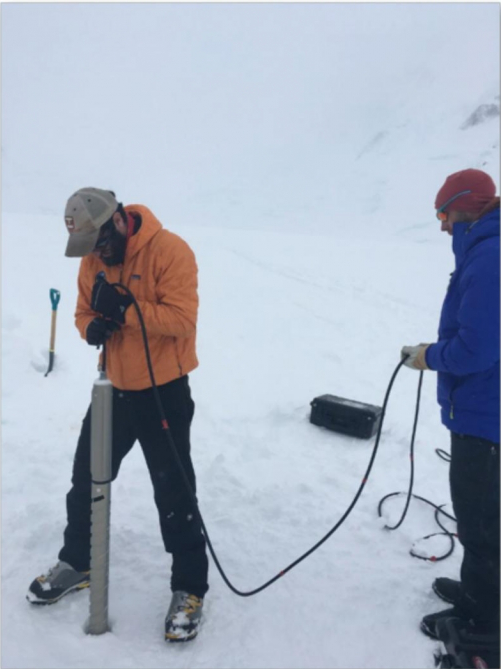
Providing the Groundwork for Future Paleoclimate and Glaciological Exploration on the Mt. Logan, Canada
Mt. Logan, St. Elias Range, Yukon, Canada
Field Team: Aaron Chesler1,2, Karl Kreutz1,2, Seth Campbell2,3, Adam Toolanen4
1 Climate Change Institute, 2 School of Earth and Climate Sciences, University of Maine Orono, 3 University of Washington, 4 Field Assistant
May – June 2017
Project Rational
Each Pacific El Niño Southern Oscillation (ENSO) event has globally reaching effects (Rodriguez-Fonseca et al., 2016 and references within). During an ENSO event, Pacific tropical waters undergo changes in sea surface temperatures and sea level pressures, which in turn affects normal atmospheric patterns (Rodriguez-Fonseca et al., 2016 and references within). Therefore, with increasing evidence for a currently changing climate (IPCC, 2014), it is important to understand how ENSO events effect atmospheric conditions throughout the globe. To understand these effects on the environment, we first must research the geological and paleoclimatic records to make accurate predictions for future climate models. Measuring paleoclimate records can be done through ice core research which can yield both direct and indirect records of previous atmospheric conditions. Ice cores provide direct atmospheric conditions by trapping gas concentrations and providing indirect measurements using proxies. Two proxies that are widely used are δ18O and δ2H, which record precipitation and temperature conditions.
Most ice cores are taken in polar regions (ie., Antarctica and Greenland) because the internal stratigraphy of those glaciers yield easily interpreted stratigraphic data (Cavitte et al., 2016). Mountain glaciers are generally less favorable in ice core research because they are subjected to higher rates of internal deformation (Campbell et al., 2012). If a core was used from a mountain environment, it would be difficult to know if the ice was still layered sequentially, which could drastically alter data and lead to incorrect chronological paleoclimate analysis. With improving geophysical methods (ie., ground penetrating radar and global positioning systems; GPR and GPS, respectively), we can see the extent of internal deformation with regards to the core and make the appropriate adjustments to our interpretations (Campbell et al., 2012). If cores were taken from mountain ranges using geophysical methods, then an intimate record could be identified and studied to understand the full effects throughout the course of an ENSO event.
Scientific Goals
An area of interest in the study of ENSO paleoclimate is the initiation and rapid change between the Medieval Warm Period (MWP, 800-1300 AD), and the Little Ice Age (LIA, 1300-1850 AD). Although the MWP and LIA were geologically recorded globally, their initial climate signals are relatively unknown and could have been caused by offsets in regional conditions. The current hypothesis regarding the initiation of the MWP and LIA is that there were longstanding ENSO (ie., La Nina and El Nino, respective) conditions throughout each event (Cobb et al., 2003). During an El Nino event, the eastern equatorial Pacific warms, yielding higher evaporation rates. The reverse happens with La Nina events where the eastern equatorial Pacific cools (leading to higher precipitation rates). By studying ice cores that reflect the changing conditions in the Pacific during the MWP and LIA, we can interpret paleoclimate changes on an annual scale and understand the dynamics of the atmosphere. These periods also demonstrate rapid short term (decadal) global climate fluctuations from warm to cold periods. By studying these rapid anomalous climate changes we can make more accurate predictions for future climate changes (Mann et al., 2008).
Mt. Logan Research
The ice core drilling project at Mt. Logan, St. Elias Range, Yukon, Canada was designed to study these paleoclimate changes that occurred in the Pacific Ocean leading to the MWP and LIA. Although this research is occurring in the northern Pacific, current data show the dynamics of the northern Pacific have a major impact on global climate (Di Lorenzo et al., 2008). The overarching goal of this project is to understand rapid oceanic and climate changes through the precipitation records.
This field season was the last of a larger project encompassing previously completed field work on Mt. Hunter and the Eclipse Icefield. In the ongoing research, ice cores have been collected from each of the field locations (Mt. Hunter, Mt. Logan, and Eclipse Icefield), however, because the cores were collected without geophysical data, it is difficult to know if internal stratigraphy was maintained throughout the drill site. Therefore, our goal is to take geophysical measurements in order to make the appropriate adjustments to the data based on the extent of deformation. This will be the first use of geophysical measurements across different core locations used in order to correlate ice cores. The integration of geophysical methods with ice core data allows for the expansion of paleoclimate research into remote mountainous regions where internal deformation of glacial stratigraphy may have occurred. With knowledge of internal deformation, researchers will be able to accurately assess the data with less interpretation error.
Field Work
Travelling to Base Camp
Adam and I flew into Whitehorse on the 20th of May. Dr. Kreutz and Dr. Campbell were already on the Eclipse Icefield with another research crew (see Understanding Paleo-Climate, Modern Climate, and Glacier Dynamics in Western Canada: Field Report from Eclipse Ice Field; http://climatechange.umaine.edu/eclipse_ice_field_expedition__2017). While in Whitehorse, Adam and I bought food supplies and any last-minute items needed for the field before heading 3 hours north to Kluane Research station, which is run by the University of Alberta. There we had beautiful views of the Kluane National Park and Reserve. When the weather cleared Adam and myself flew up to meet Dr. Kreutz and Dr. Campbell who were already on the Quinta Sello Glacier (Figure 1 and 2).
Upon arriving we spent the first few days at basecamp (Figure 3) acclimatizing and waiting for the weather to clear up-glacier. When the weather cleared we moved up to 11,500ft. There we set up a more permanent base camp. We received our helicopter support, which brought some of the heavier equipment, such as the GPR up. We then headed up to the King Trench to King Col where we took a GPR profile with the 100 MHz radar and dug a small (~1m snow pit) to take isotope samples (Figure 4).
The hike up to King Col took about 8 hours of up glacier travel but the ski down only took about 45 minutes! We had a good night sleep and the next day took a 13.5m ice core in the King Trench (Figure 5). Due to the local accumulation rate, the ice core chronicles the previous few years and will help evaluate recent changes in the North Pacific atmosphere and will help establish how local pressure changes react to ENSO variability.
Travelling off the ice
After taking our 13.5ft core at 11,500ft we waited for the next available clear day and transported some of the science gear down to 9,500ft. After establishing a cache site, we headed back up the glacier to 11,500ft for one or two more days of cloudy weather. We then packed up camp and made our final decent to 9,500ft. There we waited a couple more days and we were eventually picked up by Icefield Discovery Tours. Upon touching down at Kluane Research Station, Dr. Kretuz and I transferred ice core samples taken both from the snow pit at King Col and the core at King Trench into vials that prevent changes in isotope concentrations. These samples will be analyzed here at the Climate Change Institute over the next few months!
Acknowledgements
I would like to thank the Dan and Betty Churchill Exploration Fund for supporting travel and field logistics. I would also like to thank Dr. Karl Kreutz and Dr. Seth Campbell for giving me the opportunity to work alongside them in the field. This field expedition was also supported by a P2C2 NSF Grant Number 1502783. Thank you to Icefield Discovery and the University of Alberta Kluane Research Station Staff.
Work Cited
Campbell, S., Kreutz, K., Osterberg, E., Arcone, S., Wake, C., Introne, D., Volkening, K., and Winski, D., 2012, Melt regimes, stratigraphy, flow dynamics and glaciochemistry of three glaciers in the Alaska Range: Journal of Glaciology, v. 58, no. 207, p. 99-109.
Cavitte, M. G. P., Blankenship, D. D., Young, D. A., Schroeder, D. M., Parrenin, F., Lemeur, E., Macgregor, J. A., and Siegert, M. J., 2016, Deep radiostratigraphy of the East Antarctic plateau: connecting the Dome C and Vostok ice core sites: Journal of Glaciology, v. 62, no. 232, p. 323-334.
Cobb, K. M., Charles, C. D., Cheng, H., and Edwards, R. L., 2003, El Nino/Southern Oscillation and tropical Pacific climate during the last millennium: Nature, v. 424, no. 6946, p. 271-276.
Di Lorenzo, E., Schneider, N., Cobb, K. M., Franks, P. J. S., Chhak, K., Miller, A. J., McWilliams, J. C., Bograd, S. J., Arango, H., Curchitser, E., Powell, T. M., and Riviere, P., 2008, North Pacific Gyre Oscillation links ocean climate and ecosystem change: Geophysical Research Letters, v. 35, no. 8.
IPCC, 2014, Climate Change 2014: Synthesis Report. Contribution of Working Groups I, II, and III tot he Fifth Assessment Report of the Intergovernmental Panel on Climate Change, inCore Writing Team, R. K. P. a. L. A. M. e., ed., IPCC: Geneva, Switzerland, p. 151 pp.
Mann, M. E., Zhang, Z. H., Hughes, M. K., Bradley, R. S., Miller, S. K., Rutherford, S., and Ni, F. B., 2008, Proxy-based reconstructions of hemispheric and global surface temperature variations over the past two millennia: Proceedings of the National Academy of Sciences of the United States of America, v. 105, no. 36, p. 13252-13257.
Rodriguez-Fonseca, B., Suarez-Moreno, R., Ayarzaguena, B., Lopez-Parages, J., Gomara, I., Villamayor, J., Mohino, E., Losada, T., and Castano-Tierno, A., 2016, A Review of ENSO Influence on the North Atlantic. A Non-Stationary Signal: Atmosphere, v. 7, no. 7, p. 87.